Crash Course
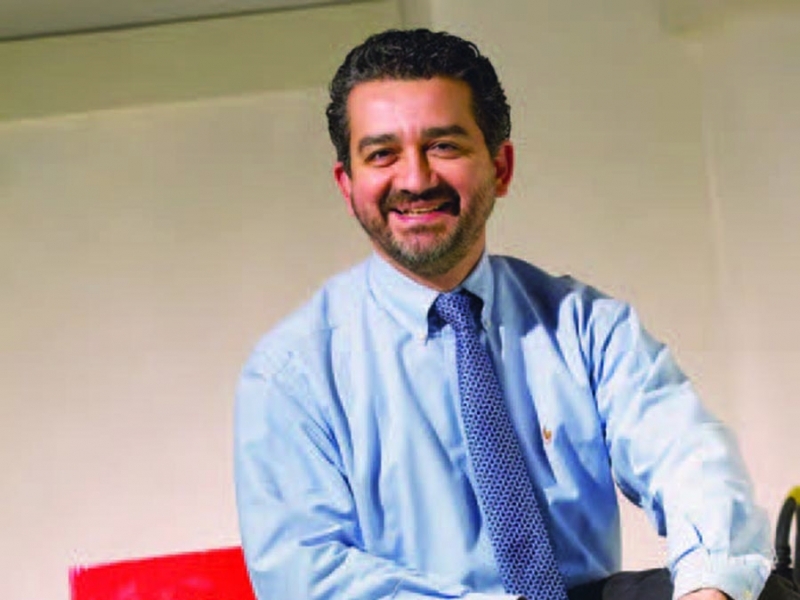
Story by Anna Nguyen
Illustration by Lynda Cloud-Weber
A screech fills the air as a vehicle begins barreling down a track at almost 40 miles an hour. The vehicle careens swiftly before slamming to a halt, all in a fraction of a second.
After the impact, a man approaches the vehicle to assess the damage inside. But the accident did not take place at a crash-test site; it happened on Main Campus, in a lab in the College of Engineering, on a small-scale crash simulator sitting atop a 19-foot aluminum track that was constructed to examine one of the most common, yet misunderstood, motor-vehicle-related injuries: traumatic aortic rupture (TAR).
Traumatic aortic rupture—a partial or complete tear in the aortic wall—is the second-leading cause of death from motor-vehicle accidents, with 12 to 29 percent of vehicular deaths related to it, according to studies by the Indiana University School of Medicine in Indianapolis and the London Health Sciences Centre in Ontario, Canada, among others. (Traumatic brain injury is the leading cause of deaths related to car accidents.)
In the U.S. and Canada, TAR results in 7,500 to 8,000 deaths annually. And as researchers in the College of Engineering note, those figures have remained constant for the past 60 years—even with the use of seatbelts and airbags being widespread.
It takes only 30 milliseconds for TAR to occur during a crash. In the Biomechanics Laboratory, the car that just crashed is a clear, 12-inch by 6-inch polycarbonate rectangular box containing a porcine aorta filled with water. When the vehicle collides with a shock absorber, the aorta continues moving into an indenter—a piece of metal that pinches the aorta to recreate TAR.
Replicating that injury is one part of the research led by Kurosh Darvish, director of the Biomechanics Laboratory and an associate professor in the College of Engineering. A leader in his field, Darvish aspires to change TAR´s persistent fatality rates by using biomechanics, an innovative field that applies mechanics and mechanical engineering principles to health-related issues.
Darvish wants to examine the injury in young and healthy tissue, because it has not yet been altered by age and is less likely to have been changed by disease. When more is understood about TAR in young, healthy tissue, researchers can examine how it affects tissue that has aged already. Under what conditions is the risk of aortic injury higher? Will it lead to an aneurysm? Catastrophic failure? Will it be able to heal on its own?
This is what researchers do know: The aorta is the body´s largest artery, and carries blood from the heart to the rest of the body. It contains four sections: the ascending aorta, the aortic arch, the descending thoracic aorta and the abdominal aorta. As the blood travels from the heart, the ascending aorta moves up through the chest toward the head. Then, it bends or arches and reaches the descending aorta, which moves down through the chest and abdomen. When TAR occurs, the majority of tears happen in the aortic isthmus, the point where the mobile aortic arch meets the more fixed descending aorta, attached to the chest wall.
Exactly how the aorta tears after the chest hits a steering wheel or an airbag is unknown, but researchers suspect the cause involves a complex combination of forces following that blow: the blood pressure rising from the impact, the upward movement of the heart and the pinching of the aorta between the front of the chest wall and the spine.
If not treated quickly, TAR can cause profuse bleeding that can result in death. It has an 85-percent mortality rate at the accident scene. When it is not immediately fatal, the condition is difficult to detect and may go unnoticed, since most patients do not exhibit symptoms. Of those who survive the initial injury, a majority will die from its worsening over time.
"We need to understand how the failure happens," Darvish says. "There is a progression of injury. It´s not just injury and no injury. We want to know when the injury becomes a problem and needs medical intervention. We want to be able to predict if it needs treatment, from surgery to medication."
In the medical world, clinicians could predict the probability and severity of TAR to determine optimal treatment options. In the commercial setting, automakers can use those answers to aid in designing more effective safety devices, such as seatbelts and airbags.
ON IMPACT
Tissue-biomechanics researchers like Darvish seek to find mechanical measures to predict failure in tissues. Working with tissue presents a challenge, since it does not all behave the same way in experiments and has many components.
Researchers use mathematics to combat those complexities and analyze the damage or deformation of the tissue—the change in its shape or size due to an applied force. The tissue´s composition changes significantly when it becomes damaged from stress and strain, Darvish says.
Funded in part by grants from the National Institutes of Health, Darvish and his team of seven graduate students—one master, six doctoral—develop and use physical and computer models to replicate TAR. They analyze how applied force, pressure and acceleration affect the severity of the injury.
Darvish also works with collaborators in the School of Medicine—Professor of Physiology Michael Autieri, CHPSW ´85, and Associate Professor of Surgery and Chief of Vascular Surgery Eric Choi.
"We needed to find simple and repeatable ways to test tissue," Darvish explains. "Based on that, we can make a computer model. What we don´t have is a model of how the tissue fails."
For the physical model, Darvish uses the aluminum track and vehicle to simulate a car crash precisely. Video, shot at 2,000 frames per second, and high-speed photography track the movement of the aorta. The aorta itself also is outfitted with photo targets that measure the biomechanical inputs. The indenter is painted black, to show its impact on the aorta.
After each crash, samples from the aorta are flash frozen with liquid nitrogen so researchers can microscopically examine thin, cross-sectional slices for tears inside the aortic wall. (The aorta tissue is approximately 1 to 2 millimeters thick.)
Large tears can be seen by the naked eye, but the microscope reveals small tears buried within layers of tissue. Darvish says that even when an aorta is not ruptured, the tears point to the possible long-term effects of an accident. Researchers then try to determine if the microscopic damage will lead to a tear or rupture of the artery, or if it can repair itself.
The physical model can create tissue failure in a controlled and replicable way to verify data from the computer model. Darvish says that many mechanical parameters—such as measures for tissue failure—cannot be measured physically, so a computer model calculates those parameters. Measurements help researchers estimate the motion and deformation of the aorta in a computer model. Darvish notes that once they can produce a similar motion using a computer model, more complex accident situations can be produced virtually. "We don´t want to alter the tissue by attaching too many sensors to it," Darvish explains. "Therefore, we make some measurements, validate the computer model against these measurements and then rely on the computer model for measurements we can´t make. We can´t measure deformation inside the aortic wall, but we can calculate it."
To help create more uniform parameters, Darvish uses math to analyze how the tissue has been changed from the impact, the variation in different layers of the aorta wall and the directional orientation of fibers within each layer of the aorta.
What he learns about the tissue also can be applied to cardiovascular diseases, such as the loss of the elastic properties of a blood vessel due to disease or aging. In the case of the aorta, that loss can cause the separation and tearing of layers of the aortic wall.
ON THE ROAD
"The work [Darvish] does is very unique and important," saysMohammad Kiani, professor and chair of mechanical engineering, and director of the Biofluidics Laboratory in the College of Engineering. "With accidents in general, we want to understand how injury impacts the human body. Then we can develop technologies to prevent them." Kiani recruited Darvish to come to Temple eight years ago.
Darvish began examining TAR in 2000, when he served as a research assistant professor of mechanical engineering at the University of Virginia in Charlottesville. The National Highway Traffic Safety Administration (NHTSA) identified TAR as an important safety issue and funded a project at that university to determine its mechanisms. Darvish says the NHTSA relies on the findings from impact-biomechanics labs to determine the safety of cars.
In the Biomechanics Laboratory, Darvish also studies traumatic brain injury, the leading cause of death from motor-vehicle crashes. Information about the deformation of brain tissue that is the result of head trauma can help spur new tools with which to measure brain injury and develop better protective systems.
Darvish´s latest work involves blast injuries suffered by soldiers. An explosion consists of three parts: a pressure wave, the projectile and a final wind. He has designed a model called a "shock tube" that examines how the first and last components of explosions can cause damage to the brain. And in the Virtual Environment and Postural Orientation Lab, the underlying causes of instability are studied in the College of Health Professions and Social Work, in order to help create therapies that will improve balance in impaired populations, such as those who have suffered from strokes.
"The more we know about traumatic injuries, the better we can prevent them and find ways to treat them more effectively," Darvish says. "The fact that my research results in saving lives excites me the most."
Anna Nguyen is a Philadelphia-based freelance writer whose work has appeared in the health section of The Philadelphia Inquirer, on WebMD and elsewhere. She also is a former reporter for two publications in New Jersey.